Magnetic resonance imaging
of the fetal brain
Annick Sévely and Claude Manelfe
Chapter Contents
- Introduction
- Safety issues
- Developments
- MR sequences
- Normal development
- Fetal cerebral abnormalities
- The present
- The future
- Summary
- References
Introduction
Fetal magnetic resonance imaging (MRI) is still in its infancy but as MR systems have become more widely available it is now the technique of choice when ultrasonography (US) detects abnormalities of the fetal brain and further elucidation is required. MRI is more sensitive than US because of its higher spatial resolution, contrast abilities and multiplanar acquisition. The first description of MRI during pregnancy was published by Smith et al. in 198325. At that time the potential of MR was limited by the long acquisition time, fetal motion and maternal respiratory artifact3, 21, 22, 28The development of ultrafast imaging sequences such as fast spin echo (FSE), half-Fourier single-shot FSE, one side image reconstruction techniques, and echoplanar imaging now allow a clinically useful MR study of the fetus without the use of sedation15, 17. T1 weighted sequences are less satisfactory than half-Fourier FSE techniques because of degradation from motion artifact, although they may be useful for confirming the presence of hemorrhage or fat.
< prev | top | contents | next >
Safety issues
Although there are no documented ill-effects to the fetus23, 27, current guidelines recommend that, as dividing cells in the embryo are more susceptible to many forms of injury, the use of MR in the first trimester of pregnancy should be avoided in normal volunteers17, 27. In addition, at the present time the quality of images below about 17 weeks’ gestation is limited by fetal size. Gadolinium-based contrast material has been used without ill-effect during pregnancy18. Gadolinium crosses the placenta and passes to the fetus. It is excreted from the fetal bladder into the amniotic fluid and is then swallowed. This potential reabsorbtion by the fetus means that the half-life of gadolinium-based contrast agents may persist for extended times. Gadolinium has been safely used in preterm infants ex utero. It is usually not necessary to monitor the fetal heart during scanning. Imaging times are also very short. However, with the development of techniques that could be used to monitor and investigate the stressed fetus or the fetus during labor, monitoring may become necessary. This could be achieved with modifications to standard Doppler ultrasound24.
< prev | top | contents | next >
Developments
Most fetal imaging is performed in the routine adult body coil. The use of arrays of surface coils should improve image quality. Vaginal ultrasound now allows good quality images of the fetus at very early stages of development and it may be mimicked in the future by the construction of internal coils for per vaginal MRI of the fetus. There has been little development of either sequences or coils that are specific for fetal imaging and there are likely to be many advances that improve signal to noise and hence the quality of images over the next decade.
< prev | top | contents | next >
MR sequences
The images used to illustrate this chapter were all acquired on a 1.5 T (Magnetom Vision, Siemens, Erlangen, Germany) with a phased-array surface coil using a HASTE sequence (half-Fourier single shot turbo spin echo)14, 16, 29 This sequence uses a 90° excitation pulse, followed by an echo train of up to 128 refocusing pulses. The half-Fourier reconstruction algorithm is used to generate a spin echo image with a matrix of 240 x 256 in 2s. A strongly T2 weighted image is obtained with TR = 10.9ms and TE = 87ms.
Five images are acquired in 10s in the axial, sagittal and coronal planes using a field of view of 400 x 400mm and a slice thickness of 5mm. For T1W imaging we use a FLASH 2D sequence, TR = 158 ms, TE = 4.8 ms with a matrix of 256 x 107, one acquisition and 5 slices of 5 mm. Total acquisition time is 17s. In the event of motion artifact the sequences may be repeated. Total examination time is usually about 5min.
Patients are usually referred because of concern over fetal brain development on antenatal ultrasound. This usually takes the form of ventricular dilation or possible agenesis of the corpus callosum.
< prev | top | contents | next >
Normal development
Maturation of the normal fetal brain may be compared to the anatomic atlas of Fees-Higgins and Larroche5 (Fig. 15.1). MRI can be used to study brain maturation9, fetal brain cellular migration7 and cortical development which is often delayed in fetuses with CNS abnormalities13. The images produced are comparable with those taken ex utero in very preterm infants (see Chapter 3). Studies of formalin-fixed embryos and fetuses give some indication of the development of different brain structures which may be used as a guide for in vivo imaging of the very immature brain20. The task for radiologists involved in MR imaging of the fetus is to know which appearances are within the normal range. This can only be ascertained by serial imaging with long-term follow-up of the infants following delivery. Several groups have noted that some ventricular asymmetry is frequently seen in both fetuses and preterm infants. The left posterior horn is slightly bigger than the right in the majority of cases. In addition, it is clear that fetuses and preterm infants up to approximately 32 weeks’ gestation have a large posterior extracerebral space which decreases with increasing gestation8. This appears to be a normal developmental feature rather than an external hydrocephalus. The evolution of cortical folding may be shown antenatally and postnatally. The maturation of the cortex may be influenced by factors such as sex, intrauterine growth retardation and multiple pregnancy. Postnatal studies suggest that there may be a 2-week individual variation between infants (see Chapter 3). Visual analysis is sufficient to identify major abnormalities of cortical development but is insufficient for identifying small differences in folding. A comparison of in utero and ex utero brain development using computer quantification (see Chapter 3) would enable us to address important issues on the role of extrinsic (ex utero) factors on brain development in the infant born prematurely.



Fig. 15.1 Normal fetus at 26 weeks’ gestation. (a) Sagittal T2 weighted image (HASTE) shows a low signal intensity corpus callosum (long arrow) and small vermis (short arrow). (b) Axial T2 weighted image (HASTE) shows large ventricles, open Sylvian fissures (long arrow), a low signal intensity cortical ribbon and normal germinal matrix over the caudate head (white arrowhead). (c) Coronal T2 weighted image (HASTE) shows normal smooth cerebral surface and a septum pellucidum cyst (arrow).
< prev | top | contents | next >
Fetal cerebral abnormalities
VENTRICULAR DILATION
The most frequent indication for fetal MRI is ventriculomegaly detected by ultrasound. MRI may be used to identify any underlying abnormalities in the brain associated with the dilated ventricles. Abnormal size and shape to the ventricles may arise as a congenital malformation (see Chapter 11), as a result of obstruction to the flow or absorption of cerebrospinal fluid, or as a result of brain atrophy. It is important to differentiate ‘normal’ ventricular asymmetry from unilateral dilatation associated with a porencephalic cyst. The increased resolution of MR may be useful to ascertain the presence of a normal regular ventricular outline and the presence of old hemorrhage. Intraventricular hemorrhage is also easily depicted by MRI because of the sensitivity of MR to the presence of blood (Fig. 15.8) (see Chapter 9). Antenatal fetal hemorrhage may be secondary to fetal clotting abnormalities, the presence of which would alter the management of the pregnancy and delivery.
In many cases, MRI is able to confirm the presence of an otherwise normal structure to the brain in the presence of ventricular dilatation (Fig. 15.2).

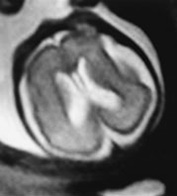
Fig. 15.2 Ventricular dilatation at 27 weeks’ gestation. (a) Coronal T2 weighted image (HASTE) shows ventricular dilatation with a cavum septum pellucidum but no other abnormalities. (b) Axial T2 weighted image (HASTE) shows the asymmetrical ventricular dilatation.


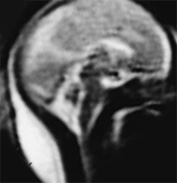
Fig. 15.3 Callosal agenesis at 25 weeksí gestation. (a) Coronal T2 weighted image (HASTE) shows absence of corpus callosum (black arrow) with continuity between the inter hemispheric fissure and ventricular system. The normal germinal matrix can be visualized as low signal intensity (white arrow). (b) Axial T2 weighted image (HASTE) shows dilated posterior portion of one lateral ventricle. (c) Sagittal T2 weighted image (HASTE): confirms the absence of corpus callosum.
AGENESIS OF CORPUS CALLOSUM
Ventricular dilation may occur with or without visualization of the corpus callosum (Figs. 15.2 and 15.3). Corpus callosal agenesis may be associated with agenesis of other commissures and with other brain malformations. These may be more easily depicted using MRI when compared with ultrasound4. An associated lipoma may be easily visualized (Fig. 15.4). The acquisition of both T1 and T2 weighted sequences will allow good differentiation of lipomatous lesions. The diagnosis of agenesis of the corpus callosum is not possible before 22 weeks’ gestation2.



Fig. 15.4 Callosal agenesis with lipoma at 34 weeks’ gestation. (a) Axial T2 weighted image (HASTE) shows splaying of the frontal horns, posterior ventricular dilatation and median low signal intensity between the frontal horns (white arrow). (b) Sagittal T2 weighted image (HASTE): there is absence of the corpus callosum and low signal intensity at the level of the genu (arrow). (c) Sagittal T1 weighted image shows the lipoma as a high signal intensity (arrow).
ARACHNOID CYST
MRI contributes in the diagnosis (Fig. 15.5) and therapeutic management of arachnoid cysts as some of these need surgical treatment after birth (Fig. 15.6). MRI is helpful in clarifying US findings in cystic malformations of the posterior fossa: Dandy–Walker malformation (Fig. 15.7), Dandy–Walker variant, vermian agenesis or arachnoid cyst or a cisterna magna (see Chapter 11, Table 11.4).

Fig. 15.5 Arachnoid cyst at 29 weeks’ gestation. Axial T2 weighted image (HASTE) shows a right well circumscribed interhemispheric cyst (arrow).

Fig. 15.6 Arachnoid cyst at 27 weeksí gestation. Axial T2 weighted image (HASTE) shows a water signal intensity extra axial cystic formation displacing the cortex. Surgical treatment was required after birth.



Fig. 15.7 Dandy-Walker malformation at 24 weeksí gestation. (a) Sagittal T2 weighted image (HASTE) shows an increase in CSF signal intensity in the posterior fossa with vermian agenesis. (b) Axial T2 weighted image (HASTE) shows hypoplasic cerebellar hemispheres (arrow). (c) Coronal T2 weighted image (HASTE) shows associated ventricular dilatation.
MYELOMENINGOCELE
MRI is useful for evaluating neural tube defects, particularly caudal abnormalities that may be difficult to detect with ultrasound. MR has been used to identify hindbrain herniation as a complication of myelomeningocele and then to monitor its evolution following antenatal closure of the neural tube defect26.
PARENCHYMAL LESIONS
Sometimes antenatal ultrasound (US) only shows ventricular enlargement and is not able to detect the associated parenchymal lesions. MRI can depict those periventricular lesions (Fig. 15.8) which have a bad prognosis and modify family counselling. Definition of white matter structures may not at present be sufficient to identify more subtle abnormalities. It is hoped that future sequence development will allow MRI to detect more subtle WM injury in the fetal brain.
TUBEROUS SCLEROSIS
The diagnosis of tuberous sclerosis is difficult with antenatal US which only shows cardiac rhabdomyoma and may not detect cerebral anomalies. MRI may improve the detection of subependymal nodules and tubers19.


Fig. 15.8 Severe periventricular leukomalacia at 35 weeks, gestation. (a) Axial T2 weighted image (HASTE) shows ventricular enlargement with periventricular high signal intensity (arrow). There is marked atrophy of the white matter and secondary abnormal cortical folding. (b) Coronal T2 weighted image (HASTE) shows the cavities in the frontal parenchyma (arrow).


Fig. 15.9 Intraventricular hemorrhage and ventricular dilatation at 34 weeksí gestation. (a) Coronal T2 weighted image (HASTE) shows ventricular dilatation with a low signal intensity consistent with hemorrhage within the choroid plexuses (arrow). (b) Axial T2 weighted image (HASTE) shows the dilatation of occipital horns, the hypointense choroid plexuses (white arrow) and a cavum septum pellucidum (black arrow).
< prev | top | contents | next >
The present
US evaluation remains the screening modality of choice to depict fetal CNS abnormalities. However, many series now report the potential of MRI to improve US diagnosis of cerebral anomalies, which may modify both the counselling of the family and the management of the pregnancy.
In our experience of all patients referred for fetal MR examinations, 25% of fetal images were normal. MRI confirmed the US findings in 50% of cases and provided additional diagnostic information in 50% of cases, which in some cases changed the management of the pregnancy.
< prev | top | contents | next >
The future
Fast MRI sequences allow an excellent study of the fetal brain without the need for sedation and already has the ability to supply valuable information about the fetus prior to delivery. This assessment could include the position of the umbilical cord, subtle abnormalities in fetal lie, and evidence of cephalo-pelvic disproportion. The future development of sequences could substantially improve the image quality, particularly for T1 weighted sequences. The additional use of contrast agents may help improve detection of lesions in certain pathological states. Perfusion/diffusion techniques could provide a unique tool for identifying areas of ischemia and for monitoring the development of white matter tracts. The acquisition of non-motion artifacted volume images will allow measurements of fetal brain structures providing normal ranges for brain development1. Subtle or new appearances on fetal brain imaging still need to be compared with the long-term clinical outcome of the child, with postnatal imaging and with histopathological study where relevant. Placental studies have been used to assess the normal and abnormal placenta6, 10 and to identify abnormal insertion of the placenta17 and MR spectroscopy may also help assess placental function in health and disease12. MR offers an exciting new tool for assessing the function of the maternal–placental–fetal unit. Functional MRI in response to auditory stimulus has been reported11. As with all functional imaging studies, the interpretation of small imaging changes in the face of motion artifact remains a problem, though not an insoluble one.
< prev | top | contents | next >
Summary
- US evaluation remains the screening modality of choice to depict fetal CNS abnormalities.
- The use of fast MRI sequences, however, does allow an excellent study of the fetal brain without the need for sedation.
- The use of MR in the first trimester of pregnancy should be avoided.
- The maturation of the normal fetal brain can be compared to the anatomic atlas of Fees-Higgins and Larroche5 and to published data on imaging in the preterm infant.
- The most frequent clinical indication for fetal MRI is ventriculomegaly detected with US with or without visualization of the corpus callosum.
- MRI contributes in the diagnosis and therapeutic management of arachnoid cysts and is helpful in clarifying US findings in cystic malformations of the posterior fossa.
- MRI can depict periventricular lesions more easily than fetal US.
- Fetal MRI is in its infancy. Coil and sequence development over the next few years should result in significant improvements in image quality.
< prev | top | contents | next >
References
- Baker PN, Johnson IR, Gowland PA et al. (1995) Measurement of fetal liver, brain and placental volumes with echo-planar magnetic resonance imaging. Br J Obstet Gynaecol 102(1), 35–39.
- Bennet GL, Bromley B and Benacerraf BR (1996) Agenesis of the corpus callosum: prenatal detection usually is not possible before 22 weeks of gestation. Radiology 199, 447–450.
- D’Ercole C, Girard N, Boubli L et al. (1993) Prenatal diagnosis of fetal cerebral abnormalities by ultrasonography and magnetic resonance imaging. Eur J Obstet Gynecol Reprod Biol 50(3), 177–184.
- D’Ercole C, Girard N, Cravello L et al. (1998) Prenatal diagnosis of fetal corpus callosum agenesis by ultrasonography and magnetic resonance imaging. Prenat Diagn 18, 247–253.
- Fees-Higgins A and Larroche J-C (1987) Le Développement du Cerveau Foetal Humain, Atlas Anatomique. INSERM CNRS Masson Paris.
- Francis ST, Duncan KR, Moore RJ et al. (1998) Non-invasive mapping of placental perfusion. Lancet 351, 1397–1399.
- Girard NJ and Raybaud C (1992) In vivo MRI of foetal brain cellular migration. J Comput-Assist Tomogr 16(2), 265–267.
- Girard N and Raybaud C (2000) Can benign hydrocephalus be recognised in utero? Child’s Nerv Syst 16, 70.
- Girard N, Raybaud C and Poncet M (1995) In vivo MR study of brain maturation in normal fetuses. Am J Neuroradiol 16 (2), 407–413.
- Gowland PA, Freeman A, Issa B et al. (1998) In vivo relaxation time measurements in the human placenta using echoplanar imaging at 0.5 T. Magn Reson Imaging 16, 241–247.
- Hykin J, Moore R, Duncan K et al. (1999) Fetal brain activity demonstrated by functional magnetic resonance imaging. Lancet 354(9179), 645–646.
- Kay HH, Hawkins SR, Gordon JD et al. (1992) Comparative analysis of normal and growth-retarded placentas with phosphorus nuclear magnetic resonance spectroscopy. Am J Obstet Gynecol 167, 548–553.
- Levine D and Barnes PD (1999) Cortical maturation in normal and abnormal fetuses as assessed with prenatal MR imaging. Radiology 210, 751–758.
- Levine D, Keedy G, Hatabu H et al. (1996) Obstetrical magnetic resonance imaging with HASTE. Proceeding SIRMS 159.
- Levine D, Keedy J, Hatabu H et al. (1996) Obstetrical magnetic resonance imaging with HASTE (abstr). Proceedings of the Fourth Meeting of the International Society for Magnetic Resonance in Medicine. Berkeley, CA, International Society for Magnetic Resonance in Medicine, 1, p. 159.
- Levine D, Barnes P, Sher S et al. (1998) Fetal fast MR imaging: reproducibility, technical quality and conspicuity of anatomy. Radiology 206, 549–554.
- Levine D, Barnes PD and Edelman RR (1999) Obstetric MR imaging. Radiology 211, 609–617.
- Marcos HB, Semelka RC and Worawattanakul S (1997) Normal placenta: gadolinium-enhanced dynamic MR imaging. Radiology 205(2), 493–496.
- Mirlesse V, Wener H, Jacquemard F et al. (1992) Magnetic resonance imaging in antenatal diagnosis of tuberous sclerosis. Lancet 340(7), 1163.
- Nakayama T and Yamada R (1999) MR imaging of the posterior fossa structures of human embryos and fetuses. Radiat Med 17(2), 105–114.
- Powel MC, Worthington BS, Buckley JM et al. (1988) Magnetic resonance imaging (MRI) in obstetrics. II. Fetal Anatomy. Br J Obstet Gynecol 95, 38–46.
- Revell M-P, Morell M-P, Bessis R et al. (1994) IRM du foetus in utero: une étude de 40 cas réalisés sans curarisation. Rev Im Med 6, 91–100.
- Schwartz J and Crooks L (1982) NMR imaging produces no observable mutations or cytotoxicity in mammalian cells. AJR 139, 583–585.
- Shakespeare SA, Moore RJ, Crowe et al. (1999) A method for foetal heart rate monitoring during magnetic resonance imaging using Doppler ultrasound. Physiol Meas 20, 363–368.
- Smith FW, Adam AH and Philips WDP (1983) NMR imaging in pregnancy. Lancet 1, 61–62.
- Sutton LN, Adzick NS, Bilaniuk LT et al. (1999) Improvement in hindbrain herniation demonstrated by serial fetal magnetic resonance imaging following fetal surgery for myelomeningocele. JAMA 17, 1826–1831.
- US Food and Drug Administration (1988) Guidance for content and review of a magnetic resonance diagnosis device 510 (k) application. Washington DC, US Food and Drug Administration, August 2.
- Weinreb JC, Lowe TW, Santos-Ramos R et al. (1985) Magnetic resonance imaging in obstetric diagnosis. Radiology 154, 157–167.
- Yamashita Y, Namimoto T, Abe Y et al. (1997) MR imaging of the fetus by a HASTE sequence. AJR 168, 513–519.