Non-accidental injury in
the young infant
Maeve McPhillips
Chapter Contents
- Mechanism of injury
- Primary injury
- Secondary injury
- Associated findings
- Differential diagnosis
- Summary
- References
Mechanism of injury
Caffey described the effects of shaking on infants, and its association with bilateral retinal hemorrhage and the typical metaphyseal corner fracture7. His theory of whiplash-shaking was supported by the finding of bilateral subdural hemorrhage, and the frequent absence of evidence of impact injury. It is true that while it is unusual to slap or spank an infant, the significance of shaking or jerking has only been realized in recent times. Shaking produces repeated acceleration– deceleration forces, so-called whiplash, mainly in an antero-posterior direction, but the brain will also rotate within the calvarium, as a secondary motion. These movements can cause tearing of the delicate bridging veins, which course from the cerebral cortex, through the subarachnoid space and the potential subdural space, to drain into the venous sinuses. This results in hemorrhage into the subarachnoid or subdural spaces (Fig.13.3b,e) or both. The infant brain is more at risk from a shaking injury due to its greater relative weight, the lack of tone in the supporting muscles of the neck, and the poor myelination associated with a higher water content. The relative degree of myelination contributes to the development of shearing injuries, most commonly at the gray–white interface, with a subcortical or callosal location. This may be a reflection of the different densities of gray and white matter.
There is often controversy as to the precise mechanism of injury, whether it be a pure shaking-whiplash injury, or whether there is an additional impact injury. The forces generated with an impact are of an order of magnitude greater than with shaking9. Impact against a soft surface, such as a mattress or sofa, does not significantly reduce the effect of the impact, but it does dissipate the trauma. Therefore the resulting signs of external injury are few, and there is no evidence of a focal impact type cerebral injury. However, it is accepted that the forces generated during a vigorous shaking alone are sufficient to cause devastating cerebral damage5. The American Academy of Pediatrics Committee on Child Abuse describes such an act as ‘so violent that competent individuals observing would recognize it as dangerous’1.
< prev | top | contents | next >
Primary injury
Primary injury is that which is caused by the trauma itself. The most subtle finding is that of subarachnoid blood. This is well described on computed tomography (CT), and has a typical location in the interhemispheric fissure posteriorly. It is recognizable as subarachnoid in location as the blood can be seen in the parasagittal sulci of the cerebral hemispheres. Recognizable blood in the basal cisterns and around the brain stem is unusual in abuse, and is more suggestive of a ruptured aneurysm. The previous relative insensitivity of magnetic resonance (MR) scanning to the presence of subarachnoid blood is one of the major reasons why CT scanning is still regarded as the most appropriate modality in the imaging of the acute phase of suspected non-accidental injury. However, with the advent of fluid-attenuated inversion recovery (FLAIR) sequences, which suppress the normal high signal from fluid in an otherwise T2 weighted sequence, small amounts of subarachnoid blood have become visible on MR16.

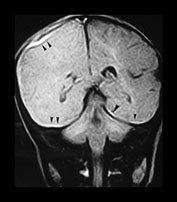
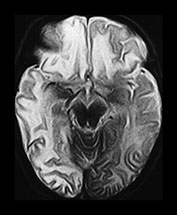



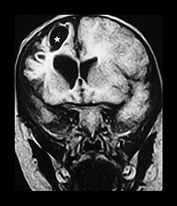
Fig. 13.1 37-day-old infant presenting with a tense fontanel and fits. All examinations performed on Picker Outlook 0.23 system. (a) T2 weighted (FSE 5100/80; FA 90°) sequence, transverse plane. Presentation scan. There is loss of the normal grayñwhite matter differentiation on the right (asterisk), sparing the frontal lobe and the occipital lobes. The left hemisphere is normal. (b) FLAIR (IRSE 3564(1600)/120 FA 90°) sequence, coronal plane. Loss of grayñwhite matter differentiation involving the right hemisphere, with some compression of the right lateral ventricle. High signal is seen over the convexity on the right and in the subtemporal region bilaterally (arrowheads), representing subdural hemorrhage. (c) T2 weighted (FSE 5100/80 FA 90°) sequence, transverse plane. Scan 10 days after presentation. There is increased intensity of the white matter of the right cerebral hemisphere, and of the frontal lobe on the left. Sulci are prominent, due to early loss of cerebral substance. (d) FLAIR (IRSE 3564 (1600)/120 FA 90°) sequence, coronal plane. There is reduced intensity of the parietal white matter on the right (stars), but the deep gray matter has been spared. There is a high intensity lesion in the right para-sagittal region (arrowhead) which appears to extend to the cortex. This is consistent with a white matter tear. (e) FLAIR (IRSE 3564 (1600)/120 FA90°) sequence, coronal plane. A more posterior image from the same examination shows a high intensity subdural hemorrhage over the right lateral parietal convexity (thick arrow). There is also increased intensity of the gyri on the right (thin arrows), and poor grayñwhite matter differentiation (star) compared to the normal left hemisphere. (f) T2 weighted (FSE 5100/80 FA 90°) sequence, transverse plane. Scan 9 months after presentation. The left cerebral hemisphere remains normal at this level. There is extensive atrophy on the right, sparing only the basal ganglia. There is white matter loss and prominence of the sulci. There is marked dilatation of the occipital horn of the right lateral ventricle. (g) FLAIR (IRSE 3564(1600)/120 FA90°) sequence, coronal plane. The previously noted white matter tear is now seen as a low intensity cystic area (star) surrounded by high signal gliosis. Again the white matter loss and atrophy of the right cerebral hemisphere is visible. The lateral ventricle is dilated. The hemicranium on the right is also smaller.
SUBDURAL HEMORRHAGE
Subdural hemorrhage is a hallmark of non-accidental shaking head injury. The blood is usually bilateral, and is described as being visible typically in the interhemispheric fissure (Fig. 13.2a). Since MR, with its multiplanar capabilities and lack of bony artifact, has become more widely used in the assessment of non-accidental injury, it is recognized that the subdural blood is usually a continuous collection and so is visible over the convexity, and in the subtemporal and subfrontal regions (Fig. 13.1b and Fig. 13.3b). Hemorrhage may be seen on either side of the tentorium, as well as along the falx. Posterior fossa subdurals may be found, although uncommonly (Fig.13.2a, b, c).
Subdural collections may show loculation, septation or fluid–fluid levels. This is often thought to imply repeated injury or hemorrhage. The evolution of a subdural hemorrhage which was uniform in intensity at initial imaging may, however, show apparent loculation with varying intensities, and the intensity may vary from anterior to posterior, or, indeed, from one side to the other (Fig 13.3d,e)15. This is particularly seen in large collections. It is therefore important for future reference and for estimating the age of the hematoma, to obtain an early scan. Ideally, this should be done before any invasive procedure such as insertion of an access device or diagnostic or therapeutic aspiration.
Much work has been done on the temporal evolution of the intensity of intracerebral hemorrhage4 (see Chapter 9). Hyperacute subdural hemorrhage may be of high, low or iso-intensity on T1 weighted images. It will be high intensity on T2 weighted images, but thin collections may not be readily visible with this sequence (Fig. 13.1a). The FLAIR sequence which suppresses the high signal of normal CSF has been of particular use in very recent hemorrhage (Fig. 13.1b). The typical acute (1–3 days) subdural hemorrhage is low intensity on both T1 and T2 or FLAIR images, evolving to high intensity on these sequences in the late subacute (1–2 weeks) phase. Unfortunately due to several possible reasons, blood in the extra-axial spaces does not always appear to behave in a totally predictable fashion (see chapter 9). High intensity may persist longer than expected (Fig. 13.2a and 13.4a). This may be because of the higher oxygen concentration in the subarachnoid space, because of layering within large collections, or because of a dilutional effect of CSF15. It may also reflect repeated bleeds, perhaps associated with therapeutic subdural aspirations. As there is rarely a precise history of the timing of the inflicted trauma in these cases, it is difficult to study the temporal evolution of the intensity of extra-axial hemorrhage.




Fig. 13.2 A 26-day infant presenting with a high-pitched cry. She had an increasing head circumference and her eyes showed ‘sunsetting’. There was occipital and facial swelling. Skeletal survey showed rib fractures and a periosteal reaction along the left humerus. (a) FLAIR (IRSE 3567(1600)/120 FA 90°) sequence, coronal plane, 24h after presentation. There is high intensity in the interhemispheric fissure on this posterior image (arrowheads), indicating subdural hemorrhage. Bilateral infratentorial low-intensity collections are also visible (stars). There was herniation of the cranio-cervical junction on the sagittal images. There is ill-defined high intensity in the subependymal regions of both lateral ventricles (arrows) suggesting hemorrhage from shearing injury. (b) T1 weighted (FE 3D 35/13 FA 40°) sequence, sagittal plane. Examination obtained 10 days after presentation. There is a low intensity posterior fossa subdural collection (star) with herniation of the cranio-cervical junction (arrows), which was seen on the presentation images also. There is a subgaleal collection over the vertex (asterisk). (c) FLAIR (IRSE 3567(1600)/120 FA 90°) sequence, coronal plane. Bilateral low-intensity collections in the posterior fossa (stars). The left sided subependymal high intensity has developed into obvious hemorrhage (arrow). (d) T1 weighted (FE 3D 35/13 FA 40°) sequence, sagittal plane. Follow-up examination obtained 10 weeks after presentation. There is a large persisting subgaleal collection (asterisk). The position of the cranio-cervical junction has returned to normal (arrow). The posterior fossa collections have resolved.
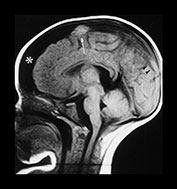





Fig. 13.3 A 6-week-old infant presenting with convulsions. Skeletal survey showed a spiral fracture of the tibia. (a) T1 weighted (FE 3D 75/28 FA 90°) sequence, sagittal plane. Presentation scan. There is widening of the pericerebral space (asterisk), with increasing intensity posteriorly (arrowheads), in keeping with layering of a subdural hemorrhage. In addition there is a focal area of high intensity over the fronto-parietal convexity (arrow), suggesting focal clot formation. (b) FLAIR (IRSE 3564(1600)/ 120 FA 90°) sequence, coronal plane. The increased intensity of subdural hemorrhage is seen in the subtemporal region bilaterally (arrowheads). In the right fronto-parietal region there is focal high signal (arrow), in keeping with clot, and the suggestion of a visible bridging vein (thin arrow). (c) T1 weighted (SE 3D 75/21 FA 90°) sequence, sagittal plane. Six days after presentation. This left para-sagittal image shows irregular increase in intensity posteriorly. In the fronto-parietal subcortical white matter there is ill-defined increase in intensity (arrow) representing a white matter tear. (d) T1 weighted (SE 3D 75/21 FA 90°) sequence, sagittal plane. This slightly more lateral image shows the variable intensity of the subdural hemorrhage, and the constant focal high signal over the fronto-parietal surface (arrow). There is also high signal from the occipital and parietal gyri (arrowheads). (e) FLAIR (IRSE 3564(1600)/120 FA 90°) sequence, coronal plane. Clear CSF is seen as low intensity in the enlarged subarachnoid space (small arrowheads). It is seen separately from the subdural space due to the different intensity of their contents. The subdural space is of medium intensity due to the presence of blood products, with focal areas of high intensity persisting in the left subtemporal and right para-falcine locations (large arrowheads). Again the suspected bridging vein is seen (thin arrow). (f) FLAIR (IRSE 3567(1600)/120 FA 90°) sequence, coronal plane. Two months after presentation. There is generalized atrophy with dilatation of the lateral ventricles and prominence of the sulci. There is focal atrophy on the left in the region of the previous white matter tear (arrowheads). There is residual high signal in the subdural space, more so on the left.
INTRACEREBRAL INJURY
Primary injury to the cerebrum itself occurs by a contusion as a result of a focal impact, by shearing of white matter due to acceleration during shaking or deceleration on impact, or rarely by compression.
Direct impact can cause cortical contusion without evidence of fracture, with an injury at the site of impact, and a contre-coup injury on the opposing aspect of the brain. The most common sites for cortical contusions are the antero-lateral and inferior surfaces of the frontal and temporal lobes. Rarely the infant’s head may be compressed, with symmetrical contusions (Fig. 13.4). Hemorrhage associated with the contusion may show layering into different intensities, visible as a fluid–fluid level (Fig. 13.4a,b). A hyperintense margin on T1 imaging is likely to be due to the presence of methemoglobin in the wall of the hemorrhage. The long-term outcome following contusion can vary, from marked atrophy and encephalomalacia to a surprising degree of recovery from an apparently devastating hemorrhage (Fig. 13.4d).
Diffuse axonal injury (DAI) is caused by shearing of the white matter, typically at the gray–white matter junction. It can also be seen in the corpus collosum, the basal ganglia, the subependymal region and in the brain stem (Fig. 13.2a). Hemorrhagic contusion is best seen as hyperintense petechial hemorrhage on T1 images, or hypointensities on T2 images. In the absence of hemorrhage, FLAIR images show DAI as hyperintensities. Resolution is with focal or diffuse atrophy with gliosis and encephalomalacia.
White matter tears are well recognized, and well seen on ultrasound12. These are seen in the anterior parietal or frontal lobes (Fig. 13.1d and Fig. 13.3c), and, again, may be hemorrhagic or non-hemorrhagic18. They probably represent a more marked shearing injury. Located in the subcortical white matter they may, rarely, extend to the cortex. Hemorrhagic tears may show fluid–fluid level formation. Healing is by cyst formation (Fig. 13.1g) or total collapse of the damaged area (Fig. 13.3f), which at autopsy may look like artifactual cleft formation.



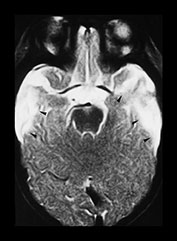

Fig. 13.4 A 6-week-old infant referred because of facial bruising. Fitting on admission. (a) T1 weighted (SE 600/15) sequence, transverse plane. Scan obtained 2 days after presentation. There is a left-sided posterior interhemispheric subdural hemorrhage seen as a high intensity stripe (arrows). There are bilateral temporal hemorrhagic contusions, with layering of blood products faintly visible within (arrowheads). (b) T1 weighted (SE 600/15) sequence, transverse plane. A more cranial image shows further areas of contusion in the parieto-occipital region bilaterally, worse on the right, where layering of blood products is visible (arrowheads). (c) T1 weighted (SE 600/15) sequence, transverse plane. Two weeks after initial scan. High signal intensity around the periphery of the bitemporal hemorrhagic contusions (arrowheads), with clearing of the central area seen as low intensity (asterisks). There is reduced signal seen in the adjacent uninvolved white matter (star) in keeping with changes of encephalomalacia. (d) T2 weighted (FSE 5100/80 FA 90°) sequence, transverse plane. Follow-up scan 7 months after presentation. There is high signal in the temporal lobes bilaterally, in keeping with cystic encephalomalacia (arrowheads), but the remainder of the brain appears remarkably normal. (e) FLAIR (IRSE 3567(1600)/120 FA 90°) sequence, coronal plane. The temporal lobes are small and atrophied (arrows) with some dark areas of cystic change.
< prev | top | contents | next >
Secondary injury
Secondary injury occurs as an indirect result of trauma. It is associated with raised intracranial pressure and may be seen as cerebral edema, diffuse brain swelling due to hyperemia, or the spectrum of hypoxia–ischemia.
EDEMA
The mechanisms by which cerebral edema occurs are not well understood. Edema is seen as a consequence of direct injury such as contusion or shearing. It has been postulated that there may be an element of strangulation or suffocation associated with the inflicted injury, but this cannot be substantiated. Vascular occlusion, arterial or venous, may occur due to entrapment of vessels by herniation.
Diffuse brain swelling is associated with rapid neurological deterioration to a Glasgow Coma Score (GCS) of 3–5. Despite being labelled as diffuse it is frequently confined to one hemisphere. It does not seem to be due to edema, or increase in water volume, as it has been shown on CT not to be associated with a decrease in density6. The swelling is therefore due to an increase in blood volume due to increased blood flow, or hyperemia. Bruce demonstrated increased cerebral blood flow in six patients with diffuse cerebral swelling, which reverted to normal when the swelling was no longer visible. How this comes about is unclear. It is possible that it is a reactive hyperemia in response to an episode of reduced perfusion or prolonged fitting. Trauma to the brain stem may produce chemical mediators which cause changes in capillary permeability. Defective autoregulation is unlikely to be responsible, as there would be accompanying raised systemic blood pressure in the presence of the raised intracranial pressure in these infants. If the injured infant survives the initial stage of diffuse brain swelling, a second stage of edema is seen some 3 days later. This then progresses to loss of cerebral bulk, with enlarged extra-axial fluid spaces and apparent atrophy. In up to 43% there is possible recovery of brain substance, perhaps as denatured myelin is reconstituted and brain protein is replenished6.
HYPOXIA–ISCHEMIA
The mechanism of the development of cerebral hypoxia– ischemia in the abused infant is not clear. It is postulated that the crying infant may be shaken into silence, which in fact is a period of apnea. Other factors may include deliberate strangulation or suffocation, or prolonged squeezing of the chest. The presence of rib fractures would be evidence for the latter. Gray matter and areas of active metabolism, such as active myelination, seem to be particularly at risk from hypoxia–ischemia.
MR findings of swelling, edema or hypoxic–ischemic change may be immediately visible or may be delayed. Diffuse cerebral swelling, which may be unilateral or bilateral, is seen as reduction in size of CSF spaces, with compression of the lateral ventricles being most obvious. Depending on the stage of evolution, gray–white matter differentiation may be lost (Fig.13.1a,b). In the presence of edema and hypoxia–ischemia, gray matter will be hypointense on T1 weighted images, and hyperintense on T2 and FLAIR images, in keeping with an increased water content.
As early as 1 week after the initial insult, some infants with hypoxia–ischemia develop a pattern of subcortical hyperintensity on T1 weighted images, which is hypointense on T2 weighted images. The changes may persist for months, or may disappear within weeks. These findings have also been described on CT where the density may suggest calcification19 but hemorrhagic change with hemosiderin formation could also account for the appearance8, 13.
In the longer term, cerebral atrophy becomes evident. There is great variability in the timing of its appearance, with earlier loss of brain substance associated with greater severity of the initial insult (Fig 13.1d, 13.3f and 13.4e). There is widening of the extra-axial spaces, with increased prominence of the sulci, widening of the subarachnoid space and dilatation of the ventricles (Fig 13.1e,f). Areas of previous hypoxic–ischemic change may be hyperintense on T2 weighted images, but hypointense on T1 and FLAIR images, in keeping with the changes of cystic encephalomalacia. Focal areas of porencephaly may also be seen where localized damage has resulted in cyst formation which may become confluent with the lateral ventricle.
< prev | top | contents | next >
Associated findings
Retinal hemorrhage is found in over 70% of non-accidental head injuries in infants. Typically bilateral in location, hemorrhage may be retinal, subretinal, preretinal, along the optic nerves or into the vitreous. Other retinal injuries such as folds, lacerations or detachments may be seen. The hemorrhages are thought to be due to a sudden rise in intravascular pressure associated with an increase in intrathoracic pressure during an episode of shaking or shaking-impact. They are not, however, pathognomonic of non-accidental injury as they may rarely be seen in severe decelerating head trauma such as deceleration injuries in road traffic accidents14. Cardiopulmonary resuscitation is recognized as a cause of retinal hemorrhage, though the incidence is disputed11, 14, through the same postulated mechanism of raised intrathoracic pressure.
Intracerebral injury can occur in the absence of obvious focal head trauma. The presence of facial or cranial bruising is, however, evidence of injury. Swelling may be from impact or from hair-pulling, and result in subgaleal collections (Fig 13.2b,d). Skull fractures in non-accidental injury may be simple or complex. A simple fracture is linear, confined to one bone, well defined and usually less than 3mm in width. A complex fracture may be branching or stellate, it can cross suture lines to involve more than one bone, or the fracture line may be diastased with separation of the edges.
Rib fractures in non-accidental injury usually occur from squeezing of the chest, commonly by adult hands. Less often they can occur by direct compression, such as kneeling on the chest. The rib may break at any point in the arc, but posterior rib fractures are perhaps the most common. They occur at the head of the rib where it articulates with the vertebral body or at the tubercle which articulates with the transverse process. Lateral and anterior rib fractures are more easily seen, while fractures of the costo-chondral junction may be difficult to see, even on oblique views15. Rib fractures which are not readily evident on a skeletal survey at the time of presentation, may be seen on a follow-up survey at 10–14 days, when callus renders them more obvious. Ultrasound has been suggested as a method of identifying rib fractures which are not obvious on radiographs22.
Apart from the finding of long bone fractures which do not fit with the history provided, the classical fracture of non-accidental injury is the metaphyseal injury. This is a subtle fracture involving the metaphysis. Depending on the radiographic projection, it may appear as a corner fracture or a bucket-handle fracture. This pattern of fracture is due to flailing and twisting of the limbs during shaking, to pulling by an extremity or direct twisting of the limb. Again, a skeletal survey is needed to detect fractures, and in selected cases follow-up radiographs of suspected sites are useful to assess healing, and to aid in dating of injuries.
< prev | top | contents | next >
Differential diagnosis
Retinal hemorrhages are not pathognomonic of non-accidental injury. Goetting reports hemorrhages in 20–50% of newborns after vaginal delivery, but almost exclusively following a cephalad delivery, not breech11. He also reports an incidence of less than 1% after cesarian section. Baum found an incidence of 31% in newborns examined in the first 12h of life, but the incidence dropped markedly in those whose eyes were first examined after 12h of age, with an incidence of 15.5% in the first week2. It is generally accepted that retinal hemorrhages will have cleared within a month after delivery. Smith examined with MR a small group of full-term, vaginally delivered neonates who had documented retinal hemorrhage. None showed any evidence of intracranial injury20.
Intracranial injury may be seen following a difficult or instrumental delivery. Subarachnoid and subdural hemorrhage is recognized in both normal breech and vacuum deliveries, typically in the posterior fossa17. Intraventricular hemorrhage may also be identified. In infants who are subsequently delivered normally, maternal injury in the prenatal period may cause intracranial injury in the fetus in utero21.
Premature infants with subsequent intraventricular hemorrhage may develop communicating hydrocephalus with dilated ventricles and widening of the extra-axial spaces. It would seem likely that tearing of bridging veins would occur more readily in these circumstances, but this has not been proven scientifically15. In any case, the degree of force needed to cause intracranial hemorrhage would still be in excess of that used in normal care of infants.
Meningoencephalitis may initially present with an obtunded infant, where the possibility of non-accidental injury cannot be discounted. Widened extra-axial spaces may be identified, which on MR will show slightly increased intensity on T1 and FLAIR sequences, reflecting an increase in protein content, or the presence of inflammatory cells. Biochemical or cytological examination of the CSF may be able to distinguish meningoencephalitis from hemorrhage.
The presence of a coagulopathy may contribute to the development of subarachnoid or subdural hemorrhage, in the absence of a history of significant trauma. Again, laboratory testing should clarify the diagnosis.
Falls and accidental injuries do occur, but, in the absence of a history of severe trauma, are rarely associated with significant intracerebral injury. Subdural hemorrhage is seen following motor vehicle accidents, but is rare following falls or crush injury10. Extensive intracranial injury, when not accompanied by an appropriate history of severe trauma, should lead to the search for other corroborative evidence of inflicted injury.
Clinicians, and in particular radiologists, must not only be aware of the possibility of non-accidental injury, but search for it and alert colleagues to the possibility. It is also of utmost importance to be aware of the conditions to be considered in the possible differential diagnosis, and positively exclude them. Only in this way will children be protected both from abuse and from over-zealous protection.
< prev | top | contents | next >
Summary
- Magnetic resonance imaging of the brain is an essential part of the investigation of any infant with suspected non-accidental head injury.
- FLAIR images are recommended for the detection of acute subdural hemorrhage.
- The known patterns of abuse produce well-recognized intracranial pathology.
- Lesions in the brain may be primary or secondary.
- Evidence for pathology elsewhere in the body must always be sought to corroborate the diagnosis of non- accidental injury.
< prev | top | contents | next >
References
- American Academy of Pediatrics (1993) Shaken baby syndrome: inflicted cerebral trauma. Pediatrics 92, 872–875.
- Baum JD and Bulpitt CJ (1970) Retinal and conjunctival hemorrhage in the newborn. Arch Dis Child 45, 344–349.
- Billmire ME and Myers PA (1985) Serious head injury in infants: accident or abuse? Pediatrics 75, 340–342.
- Bradley WG (1993) MR appearance of hemorrhage in the brain. Radiology 189, 15–26.
- Brown JK and Minns RA (1993) Non-accidental head injury, with particular reference to whiplash shaking injury and medico-legal aspects. Dev Med Child Neurol 35, 849–869.
- Bruce DA, Alavi A, Bilaniuk L et al. (1981) Diffuse cerebral swelling following head injuries in children: the syndrome of ‘malignant brain edema’. J Neurosurg 54, 170–178.
- Caffey J (1972) On the theory and practice of shaking infants. Amer J Dis Child 124, 161–169.
- Close PJ and Carty HM (1991) Transient gyriform brightness on non-contrast enhanced computed tomography (CT) brain scan of seven infants. Pediatr Radiol 21, 189–192.
- Duhaime AC, Gennarelli TA, Thibault LE et al. (1987) The shaken baby syndrome. J Neurosurg 66, 409–415.
- Ewing-Cobbs L, Kramer L, Prasad M et al. (1998) Neuroimaging, physical and developmental findings after inflicted and noninflicted traumatic brain injury in young children. Pediatrics 102, 300–307.
- Goetting MG and Sowa B (1990) Retinal hemorrhage after cardiopulmonary resuscitation in children: an etiological reevaluation. Pediatrics 85, 585–588.
- Jaspan T, Narborough G, Punt JAG et al. (1992) Cerebral contusional tears as a marker of child abuse – detection by cranial sonography. Pediatr Radiol 22, 237–245.
- Jaspan T and Stevens KJ (1999) Radiological imaging of craniocerebral non-accidental injury in infancy. RAD Magazine 25(288), 66–69.
- Kanter KK (1986) Retinal hemorrhage after cardiopulmonary resuscitation or child abuse. J Pediatr 108, 430–432.
- Kleinman PK and Barnes PD (1998) Head trauma. In: Kleinman PK (Ed.) Diagnostic Imaging of Child Abuse, 2nd edn. St Louis: Mosby, pp. 296–325.
- Noguchi K et al. (1995) Acute subarachnoid hemorrhage: MR imaging with fluid-attenuated inversion recovery pulse sequences. Radiology 196, 773–777.
- Odita JC and Hebi S (1996) CT and MRI characteristics of intracranial hemorrhage complicating breech and vacuum delivery. Pediatr Radiol 26, 782–785.
- Sato Y, Yuh WT, Smith WL et al. (1989) Head injury in child abuse; evaluation with MR imaging. Radiology 173, 653–657.
- Sener RN (1993) Gyral calcifications detected on the 45th day after cerebral infarction. Pediatr Radiol 23, 570–571.
- Smith WL, Alexander RC, Judisch GF et al. (1992) Magnetic resonance imaging evaluation of neonates with retinal hemorrhages. Pediatrics 89, 332–333.
- Stephens RP, Richardson AC and Lewin JS (1997) Bilateral subdural hematomas in a newborn infant. Pediatrics 99, 619–621.
- Wischhofer E, Fenkl R and Blum R (1995) Ultrasound detection of rib fractures for verifying fracture diagnosis. A pilot project. Unfallchirug 98(5), 296–300.